A New Enzyme Design Promises Greener Industrial Processes
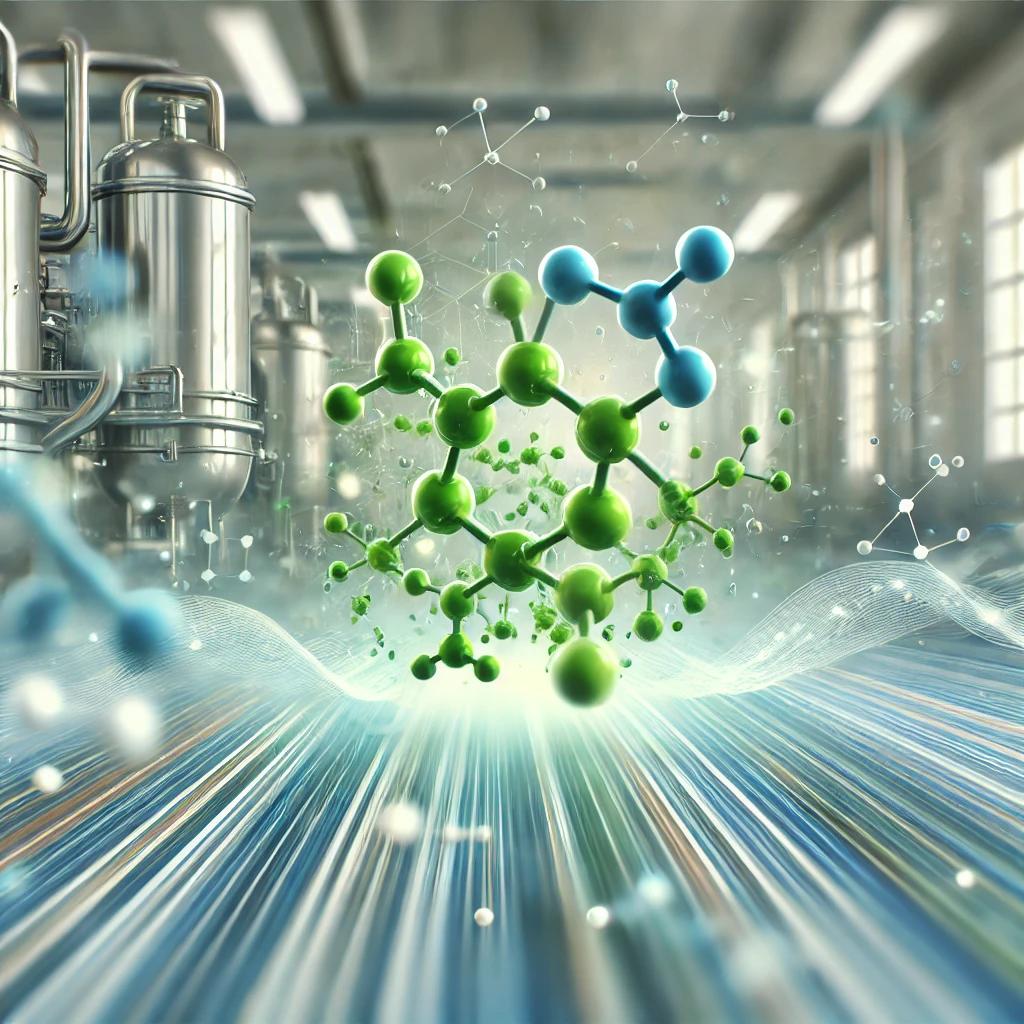
A team of researchers from Yunnan Normal University in China has unveiled a novel method to enhance the heat resistance of enzymes crucial to various industries. Their study focuses on Serine Protease PB92, an enzyme widely used in sectors ranging from leather processing to food manufacturing. By making this enzyme more tolerant to high temperatures, the researchers are paving the way for more efficient, cost-effective, and environmentally friendly industrial processes.
The Quest for a Heat-Resistant Enzyme
Enzymes are nature’s catalysts, accelerating chemical reactions that are essential to life and industry. Serine Protease PB92, derived from the bacterium Bacillus alcalophilus, is prized for its ability to break down proteins under alkaline conditions. However, its vulnerability to high temperatures has limited its application, especially in processes that require heat, such as high-temperature washing in detergents or specific treatments in textile manufacturing.
Industries have long sought enzymes that can withstand harsher conditions without losing their effectiveness. A heat-stable enzyme would not only improve efficiency but also reduce energy consumption by allowing reactions to occur at higher temperatures, where they often proceed faster.
A Clever Blend of Intuition and Technology
To tackle this challenge, the research team employed a method known as semi-rational design, a fusion of scientific intuition and computational modeling. This approach allows scientists to predict which parts of an enzyme’s structure can be modified to enhance desired properties without compromising its function.
They began by examining the three-dimensional structure of Serine Protease PB92 to identify regions that were prone to unfolding at higher temperatures. These flexible areas are like weak links in a chain, susceptible to breaking under stress. By using a parameter called the B-factor, which highlights the most mobile parts of a protein, they pinpointed specific amino acids that could be targeted for modification.
Drawing comparisons with similar enzymes from heat-loving organisms, known as thermophiles, they identified which amino acids could be swapped to increase stability. The goal was to create stronger internal bonds within the enzyme, effectively reinforcing its structure against heat-induced denaturation.
Using site-directed mutagenesis, a precise genetic editing technique, they altered the enzyme’s DNA sequence to substitute these key amino acids. This meticulous process allowed them to create variants of the enzyme with potentially enhanced heat resistance.
Promising Outcomes
The results were remarkable. Some of the modified enzymes remained stable and active even after being exposed to temperatures as high as 65°C (149°F) for extended periods—a significant improvement over the original enzyme. Importantly, these enzymes retained much of their ability to break down proteins, ensuring they remained effective for industrial use.
One standout variant combined several beneficial mutations, exhibiting both high thermostability and satisfactory activity levels. This makes it a strong candidate for real-world applications, where both durability and effectiveness are essential.
Decoding the Structural Enhancements
Going deeper into why these modifications worked, the researchers analyzed the molecular structures of the altered enzymes. They discovered that the substitutions led to increased hydrophobic interactions within the enzyme’s core. In simpler terms, parts of the enzyme became more water-repellent, which helped the molecule fold more tightly and maintain its shape at higher temperatures.
Additionally, the enzyme’s overall flexibility was reduced. While this might sound counterintuitive, a certain degree of rigidity is beneficial for stability. By locking the structure in place, the enzyme is less likely to unravel when heated. The challenge is to strike a balance where the enzyme remains stable without losing its functional ability to bind to substrates and catalyze reactions. The researchers successfully achieved this equilibrium.
Implications for Multiple Industries
The enhanced enzyme holds significant promise across various sectors:
- Detergent Manufacturing: Heat-resistant enzymes can improve the efficacy of laundry detergents, allowing them to work effectively in hot water and remove tough stains more efficiently.
- Textile Processing: In fabric production, enzymes are used for processes like bio-polishing and desizing. A thermostable enzyme can withstand the high temperatures required, leading to better-quality textiles and reduced environmental impact.
- Food Industry: Enzymes that remain active at elevated temperatures can streamline processes like brewing and cheese making, improving product consistency and potentially reducing production times.
While the laboratory results are encouraging, there is more work to be done before these enzymes can be widely adopted. Scaling up production to industrial levels will require further optimization to ensure the process is cost-effective and the enzymes remain consistent in quality.
The researchers are also interested in applying their semi-rational design approach to other enzymes facing stability challenges. By enhancing the heat resistance of a broader range of enzymes, they could facilitate advances across multiple industries, promoting more sustainable and efficient practices.
Long-term stability studies are another critical step. Assessing how these modified enzymes perform over extended periods and under various industrial conditions will be crucial for their adoption. Ensuring that the enzymes maintain their enhanced properties outside of the laboratory will determine their practical utility.
A Step Towards Sustainable Solutions
By enhancing the heat resistance of Serine Protease PB92 without sacrificing its activity, researchers have again demonstrated the power of combining structural analysis with precise genetic modifications. The ability to design enzymes that can withstand harsh conditions opens doors to more efficient industrial processes, reduced energy consumption, and a smaller environmental footprint.
As industries seek greener and more sustainable solutions, innovations like this offer a promising path forward. The fusion of biotechnology and industrial applications not only improves performance but also contributes to global efforts toward sustainability.
Reference
- Miao, H., Xiang, X., Han, N., Wu, Q., & Huang, Z. (2023). Improving the Thermostability of Serine Protease PB92 from Bacillus alcalophilus via Site-Directed Mutagenesis Based on Semi-Rational Design. MDPI.
Keywords
Serine Protease PB92, Bacillus alcalophilus, Enzyme Thermostability, Site-Directed Mutagenesis, Enzyme Engineering, Industrial Biotechnology, Protein Stability, Semi-Rational Design, High-Temperature Applications, Sustainable Processes
Disclaimer: The information presented in this article is intended for informational purposes only and reflects the findings of the referenced study as of the publication date. For detailed information, please refer to the original research paper or consult professionals in the field.